Stepper motors are installed in several household appliances, like in washing machines, in conditioners and in refrigerators, they are characterized by a low-speed and high-torque operation.
With the unceasing technology development, also motors play a fundamental role as actuators and automation elements. Stepper motors, in particular, are a kind of motor that converts electrical pulse signals into rotary motion, their operation principle is based on the electromagnetic induction. They are structurally simple, precise and easily controllable, then they are widely used in household appliances.
Stepper motors play a fundamental role in manifold domestic applications, providing household appliances with a precise and reliable motion control.
This type of motor can drive key components such as the air conditioner damper and fan, controlling its flow with the consequent adjustment of the environmental temperature through a gradual and precise motion. Stepper motors can be used also to drive key components like the spin basket and the water pump in washing machines, assisting in the precise control of the washing programme progress and the water level control.
Other applications concern small household appliances like, for instance, coffee machines and mixers.
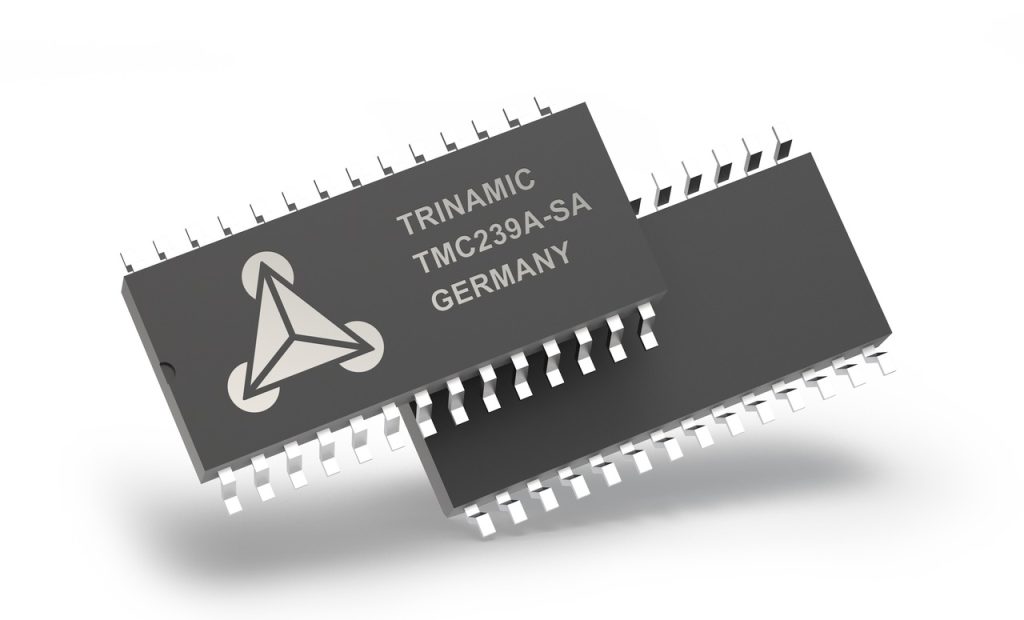
Microstepping for smooth precise motions
Stepper motors use a permanently magnetized rotor and some coils as stator. Sending an electric current through motor coils creates an electromagnetic field that forces the magnetic rotor into the wished position. A typical stepper motor has 50 pairs of magnetic poles, which allow the motor to approach the 200 steps, that is to say the 200 positions for a complete 360° revolution. However, it is possible to generate smaller steps, such as “half steps” or microsteps, using additional stators. This increases precision, torque and efficiency of the motor, meanwhile reducing the loss of steps, vibrations and noise.
In fact, stepper motor drives can be controlled with a sinusoidal current waveform, actually subdividing complete steps into smaller microsteps to achieve the maximum precision and a smooth motion.
With a maximum of 256 microsteps for a complete step, Trinamic technology by Analog Devices transforms digital information into a perfect physical motion. Drive current control technology not only decreases noise and vibrations, but at the same time it increases the attainable torque and precision.
Stepper motors are durable, open-loop motors, which offer numerous advantages in comparison with other electric motors: they have high torque both at low speeds and in stop state. Their marginal positioning error is not cumulative; they have excellent response to start/stop conditions and they are convenient and user-friendly, too. All this makes stepper motors an advantageous choice when a precise and reliable open-loop positioning is necessary. As anticipated, stepper motors operate by sending an electric current to motor coils to create an electromagnetic field that forces the magnetic rotor into the desired position: this can be executed in complete steps or with the smaller microsteps, by using additional stators and Trinamic technology.
The difference among steps, half steps and microsteps
Mechanically, a stepper motor is similar to a mass-spring system. Moving from one position to another, the rotor does not find the right position immediately, but it will oscillate around the target position until it reaches it. And precisely like a mass-spring system, the bigger the difference between the starting position and the desired one, the bigger the oscillation around the target point.
Microstepping aims at driving motors with a current waveform that approximates a sinusoid (figure 1). This means that stator coils are not powered by a current that is at the maximum value or at zero, but by intermediate current levels that on the whole approximate a sinusoidal waveform that covers four complete steps. This positions the permanent magnet rotor in intermediate positions between two complete successive phases. Moreover, the microstepping allows customized current waveforms, adapted to physics or to the particular application of the stepper motor. The maximum resolution of microsteps is defined by the analog-to digital (A/D) and digital-to-analog (D/A) capacities of the driver’s electronics.
The motion controller integrated into Trinamic cDriver products by Analog Devices exploits the microstepping technology, and integrated driver circuits offer the resolution of 256 microsteps.
If adapted to a hybrid stepper motor, the Microstep Trinamic 256× technology provides 51,200 microsteps for complete 360° rotations; this implies the availability of a precision that reaches 0.00703125° for a complete rotation.
The Trinamic MicroPlyer interpolator adds current steps among step pulses, preserving position and speed. This allows any motor control system to operate with a high resolution, offering a high-level stepper motor control without needing a complete system update.
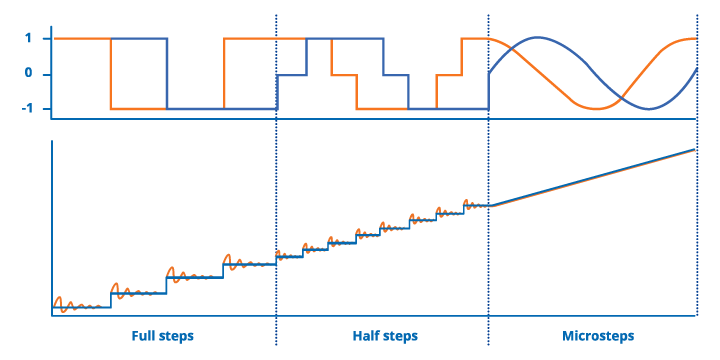
Precise, reactive, reliable and durable control
The motion control is given by the control of the position, of the speed and of the acceleration, which on the whole enable today’s highly automated physical world. Whenever an object must be in the right place at the right time, the control of its motion is needed. Since real items are displaced, the motion control technology requires a precision and a reliability that must be at least equal to many other sophisticated disciplines. Trinamic motion control technology by Analog Devices provides this high-precision solutions with high reliability, to support a multitude of sometimes unique requirements.
Trinamic ramp generators perform the motion control, freeing the microcontroller from this task. By adjusting the position, the speed and the acceleration of actuators (as electric motors are), Trinamic technology actually optimizes the motion control with ramp profiles that offer high performances for any motion control requirement, even in case of challenging applications that move more axes in synchrony one another.
The simplest way to drive a load is using a constant speed (ramp profile). Since that modality has not any defined acceleration phase, the theoretical acceleration value is infinite. However, both the system and the load have a behaviour in the finite time that must be accelerated at the requested speed. Consequently, inconstant time delays occur for the acceleration depending on the system and on the load.
Owing to the relationship between speed and distance, a precise positioning is not possible without carrying out further regulations. Moreover, if the difference between the target speed and the effective speed of the system is not too big, the motor might stall or overshoot.
Ramp profiles take into account the difference between theoretical speed ramps and the real physical world. Trinamic motion control technology offers various ramp profiles, from the trapezoidal one to the S-shaped one. The ramp generation is integrated into motion control peripherals to free, as anticipated, the processor from tasks to be executed in real time, meanwhile providing excellent performances.
Trapezoidal ramp
A trapezoidal ramp (figure 2) provides for acceleration rates by using a constant gradient. This leads to a linear increase and decrease of the system speed, therefore a constant gradient is used for the acceleration (aMAX) and one for the deceleration (dMAX) up to the maximum speed (vMAX). This constant gradient enables the precise calculation and consideration of changeable time delays. Linear ramp profiles are sufficient for the vast majority of applications with simple positioning.
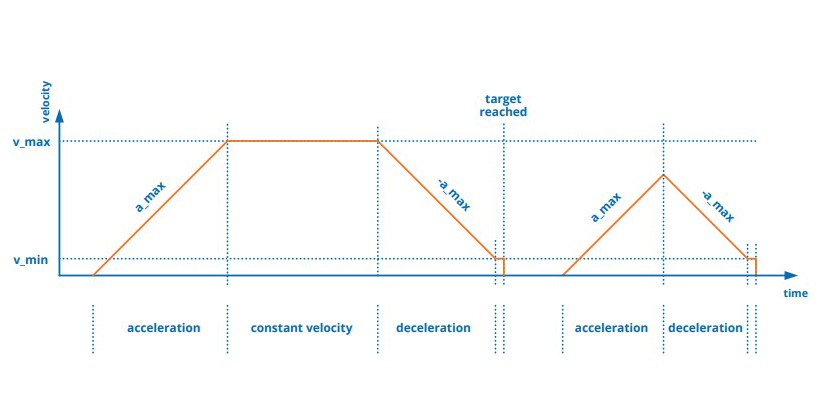
Segmented ramp
By adding a freely configurable start/stop frequency to linear motion profiles, the segmented ramp allows a much faster positioning and mitigates the resonances caused by the trapezoidal ramp (figure 3). Moreover, the profile adds a low acceleration value at high speed, which reduces jerking at the end of standard acceleration ramps. Once reached the target position, the motor waits for the TZEROWAIT clock cycle to ascertain that all oscillations have disappeared from the mechanical system, enabling a fast positioning through additional acceleration segments (figure 4).
Segmented ramps bring flexibility to the speed profile modelling, adapting it to the torque/speed curve, limiting jerking and decreasing the time-to-target for precise positioning applications.
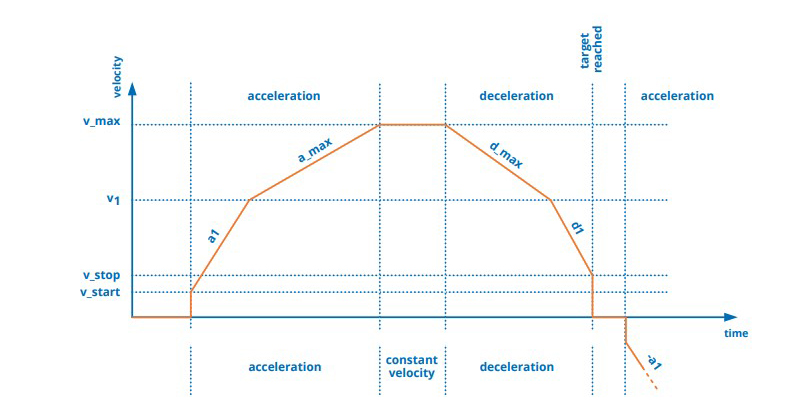
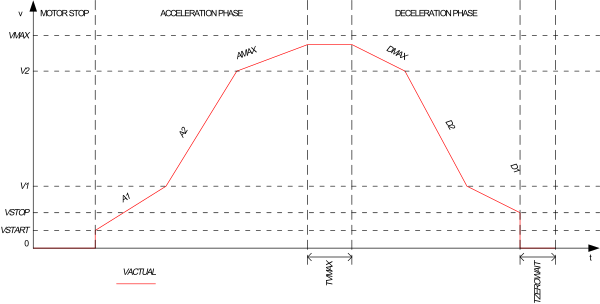
S-shaped ramp
Especially when we work with delicate devices, it is important to have a smooth motion control, avoiding the possible resonances. To hit this target, a gradual modification of the acceleration parameter is necessary (figure 5). Such gradual variation, or curvature parameter, minimizes mechanical vibrations, eliminating the motor’s overshoot problems. Moreover, it is possible to reach high torques with high speeds calibrating the arches of the speed ramp and then permitting to optimize the profile according to one’s own application. The resulting profile with continuous acceleration and deceleration reduces any sudden motion.
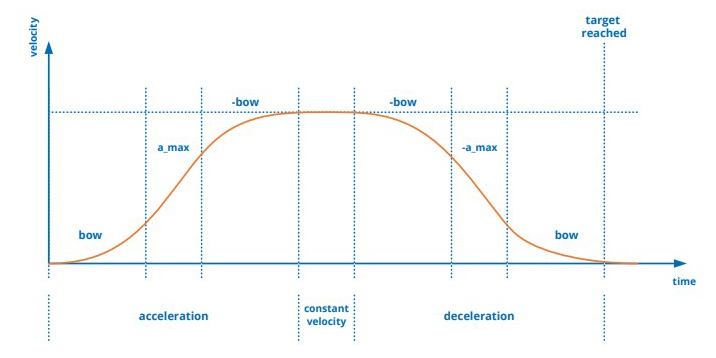